There is much more to undertand about gravity, including the search for the missing (fictional) gravity waves, notwithstanding the fact that a nobel prize has already been awarded for their discovery, but we will follow that debate on the following pages.
First we must investigate the structure of the nucleus of the atom and get it right, to become a nuclear physicist.
Gravitation, or gravity, is a natural phenomenon by which physical bodies attract with a force proportional to their mass. Gravitation is most familiar as the agent that gives weight to objects with mass and causes them to fall to the ground when dropped. Gravitation causes dispersed matter to coalesce, and coalesced matter to remain intact, thus accounting for the existence of the Earth, the Sun, and most of the macroscopic objects in the universe.
Gravitation is responsible for keeping the Earth and the other planets in their orbits around the Sun; for keeping the Moon in its orbit around the Earth; for the formation of tides; for natural convection, by which fluid flow occurs under the influence of a density gradient and gravity; for heating the interiors of forming stars and planets to very high temperatures; and for various other phenomena observed on Earth.
Gravitation is one of the four fundamental interactions of nature, along with electromagnetism, and the nuclear strong force and weak force. Modern physics describes gravitation using the general theory of relativity by Einstein, in which it is a consequence of the curvature of spacetime governing the motion of inertial objects. The simpler Newton's law of universal gravitation provides an accurate approximation for most physical situations.
Every planetary body (including the Earth) is surrounded by its own gravitational field, which exerts an attractive force on all objects. Assuming a spherically symmetrical planet, the strength of this field at any given point is proportional to the planetary body's mass and inversely proportional to the square of the distance from the center of the body.
The strength of the gravitational field is numerically equal to the acceleration of objects under its influence, and its value at the Earth's surface, denoted g, is approximately expressed below as the standard average.
g = 9.81 m/s2 = 32.2 ft/s2
This means that, ignoring air resistance, an object falling freely near the Earth's surface increases its velocity by 9.81 m/s (32.2 ft/s or 22 mph) for each second of its descent. Thus, an object starting from rest will attain a velocity of 9.81 m/s (32.2 ft/s) after one second, 19.6 m/s (64.4 ft/s) after two seconds, and so on, adding 9.81 m/s (32.2 ft/s) to each resulting velocity. Also, again ignoring air resistance, any and all objects, when dropped from the same height, will hit the ground at the same time.
According to Newton's 3rd Law, the Earth itself experiences a force equal in magnitude and opposite in direction to that which it exerts on a falling object. This means that the Earth also accelerates towards the object until they collide. Because the mass of the Earth is huge, however, the acceleration imparted to the Earth by this opposite force is negligible in comparison to the object's. If the object doesn't bounce after it has collided with the Earth, each of them then exerts a repulsive contact force on the other which effectively balances the attractive force of gravity and prevents further acceleration.
Under an assumption of constant gravity, Newton's law of universal gravitation simplifies to F = mg, where m is the mass of the body and g is a constant vector with an average magnitude of 9.81 m/s2. The acceleration due to gravity is equal to this g. An initially stationary object which is allowed to fall freely under gravity drops a distance which is proportional to the square of the elapsed time. The image on the right, spanning half a second, was captured with a stroboscopic flash at 20 flashes per second. During the first 1⁄20 of a second the ball drops one unit of distance (here, a unit is about 12 mm); by 2⁄20 it has dropped at total of 4 units; by 3⁄20, 9 units and so on.
Under the same constant gravity assumptions, the potential energy, Ep, of a body at height h is given by Ep = mgh (or Ep = Wh, with W meaning weight). This expression is valid only over small distances h from the surface of the Earth. Similarly, the expression for the maximum height reached by a vertically projected body with velocity v is useful for small heights and small initial velocities only.
The discovery and application of Newton's law of gravity accounts for the detailed information we have about the planets in our solar system, the mass of the Sun, the distance to stars, quasars and even the theory of dark matter. Although we have not traveled to all the planets nor to the Sun, we know their masses. These masses are obtained by applying the laws of gravity to the measured characteristics of the orbit. In space an object maintains its orbit because of the force of gravity acting upon it. Planets orbit stars, stars orbit Galactic Centers, galaxies orbit a center of mass in clusters, and clusters orbit in superclusters. The force of gravity exerted on one object by another is directly proportional to the product of those objects' masses and inversely proportional to the square of the distance between them.
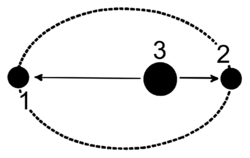
Apsides 1) Apoapsis; 2) Periapsis; 3) Focus
An apsis (Greek ἁψίς, gen. ἁψίδος), plural apsides ( /ˈæpsɨdiːz/; Greek: ἁψίδες), is the point of greatest or least distance of a body from one of the foci of its elliptical orbit. In modern celestial mechanics this focus is also the center of attraction, which is usually the center of mass of the system. Historically, in geocentric systems, apsides were measured from the center of the Earth.
The point of closest approach (the point at which two bodies are the closest) is called the periapsis or pericentre, from Greek περί, peri, around, and κέντρον kentron. The point of farthest excursion is called the apoapsis (ἀπό, apó, "from", apocentre or apapsis [from ἀπ-, ap-, before an unaspirated, or ἀφ-, aph-, before an aspirated vowel, respectively]), (the latter term, although etymologically more correct, is much less used). A straight line drawn through the periapsis and apoapsis is the line of apsides. This is the major axis of the ellipse, the line through the longest part of the ellipse.
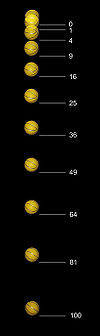
Ball falling freely under gravity. See text for description
Do you notice that the relationship between the numbers is the same series as in the square of a number?
1,- 1
2,- 4
3,- 9
4,- 16
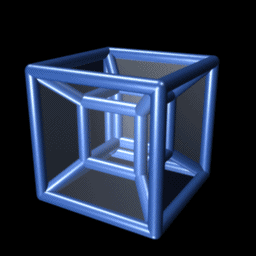
Imagine that the illustration above is an object, not expanding, but falling in on itself.
Galileo Ramp Lab
Introduction: Until the time of Galileo, everyone accepted the Aristotelian concepts of physics. Aristotle taught that everything tries to seek its “proper place”. In Aristotle's view, lighter objects fall more slowly than heavier ones, simply because lighter things contain more of the lighter elements, air and fire which tend to rise, and heavier things tend to fall faster because they contain more earth and water. Similarly, Aristotle said that the natural state of an object is to be at rest, so that an object will stop moving as soon as the force acting on it is removed. Aristotle had no concept of acceleration.
Galileo did a series of experiments to show that all bodies accelerate toward the earth and that they all accelerate in the same way. He also showed that in the absence of friction, bodies would keep moving forever. In effect, he achieved a partial understanding of gravity, and he discovered the concepts of acceleration and inertia. Galileo (and Newton after him) showed that the acceleration of gravity is a constant for all objects at the surface of the Earth, which means that the velocity of a falling object increases continuously. In Newtonian terms, Galileo found that the following relations hold:
Vf= a*t when Vi = 0
d = ½ at2 when Vi = 0
Where Vf is the final velocity of the object after falling for time, t, the acceleration is a and d is the distance the object has fallen. The average velocity (of any object) is just
Va= d / t
In this experiment, you will be able to calculate the average velocity, Va. The final velocity, Vf, will be twice as large (2Va)