We all know there is no gravity in space, don't we? We have seen pictures of astronauts floating inside the space shuttle. So what is the mystery of gravity? Why does water float out of a cup in space, but a man's arms not fly off? Even on the moon where gravity is one sixth of what it is on earth, it is enough to keep a man from flying off into space.
Einstein made some predictions regarding special relativity in a situation where there is no gravity. One of them was gravitational lensing using the mass of the sun. Photographs were taken during a solar eclipse and the predicted results were confirmed.
What he predicted was a slight but significant difference between Newtonian gravitation and General relativity (Einsteinian) gravitation.
So why does the sun (a large mass) bend the light away from itself, and not toward it? Or does it? The image of an Einstein cross, appears to clearly show four images of the same object, making one think that light is split into four beam, each passing on different sides of a far away (400 million light years) object. But the light could equally be passing through the galaxy, but passing on different sides of various large masses within the galaxy. Why four, but only four images of the distany quasar (light source)?
One might reasonably think that a mass (a photon) would be attracted toward a mass like the sun. Perhaps it is because the photon (light) actually picks up velocity when it is caught by (passes close to) the mass of the sun. It cannot be a significant amount, because the photon is already travelling at the speed of light.
This has been calculated as a blue shift as the photon is drawn toward a mass, and a red shift as it is ejected (escapes) from that orbit, and is 'slingshot' on its way at maximum velocity. Light travels in a straight line except when it passes close by a large mass. Why does light slow when it passes a mass? One would assume it would accellerate toward the mass, but perhaps it is being slowed, and that is why it blue-shifts as it approaches a mass. A subtle difference.
Can we assume that this happens with all masses? By this I mean, those like suns with strong magnetic fields, and light emissions, those with just magnetic fields (with a molten core), and those which may be solid with little or no magnetic radiation?
Einstein correctly guessed that the closest planet in the solar system (Mercury) had an orbit different from that predicted by Newtonian gravitational constants, and corrected the figures.
Einstein correctly predicted the red shift of
Assuming that the equivalence principle holds,[50] gravity influences the passage of time. Light sent down into a gravity well is blueshifted, whereas light sent in the opposite direction (i.e., climbing out of the gravity well) is redshifted; collectively, these two effects are known as the gravitational frequency shift. More generally, processes close to a massive body run more slowly when compared with processes taking place farther away; this effect is known as gravitational time dilation.[51]
Gravitational redshift has been measured in the laboratory[52] and using astronomical observations.[53] Gravitational time dilation in the Earth's gravitational field has been measured numerous times using atomic clocks,[54] while ongoing validation is provided as a side effect of the operation of the Global Positioning System (GPS).[55] Tests in stronger gravitational fields are provided by the observation of binary pulsars.[56] All results are in agreement with general relativity.[57] However, at the current level of accuracy, these observations cannot distinguish between general relativity and other theories in which the equivalence principle is valid.[58]
Light deflection and gravitational time delay
General relativity predicts that the path of light is bent in a gravitational field; light passing a massive body is deflected towards that body. This effect has been confirmed by observing the light of stars or distant quasars being deflected as it passes the Sun.[59]
This and related predictions follow from the fact that light follows what is called a light-like or null geodesic—a generalization of the straight lines along which light travels in classical physics. Such geodesics are the generalization of the invariance of lightspeed in special relativity.[60]
As one examines suitable model spacetimes (either the exterior Schwarzschild solution or, for more than a single mass, the post-Newtonian expansion),[61] several effects of gravity on light propagation emerge. Although the bending of light can also be derived by extending the universality of free fall to light,[62] the angle of deflection resulting from such calculations is only half the value given by general relativity.[63]
Closely related to light deflection is the gravitational time delay (or Shapiro effect), the phenomenon that light signals take longer to move through a gravitational field than they would in the absence of that field. There have been numerous successful tests of this prediction.[64] In the parameterized post-Newtonian formalism (PPN), measurements of both the deflection of light and the gravitational time delay determine a parameter called γ, which encodes the influence of gravity on the geometry of space.[65]
Henry Cavendish in 1784 (in an unpublished manuscript) and Johann Georg von Soldner in 1801 (published in 1804) had pointed out that Newtonian gravity predicts that starlight will bend around a massive object.[9] The same value as Soldner's was calculated by Einstein in 1911 based on the equivalence principle alone. However, Einstein noted in 1915 in the process of completing general relativity, that his (and thus Soldner's) 1911-result is only half of the correct value. Einstein became the first to calculate the correct value for light bending.[10]
The first observation of light deflection was performed by noting the change in position of stars as they passed near the Sun on the celestial sphere. The observations were performed in 1919 by Arthur Eddington and his collaborators during a total solar eclipse,[11] so that the stars near the Sun could be observed. Observations were made simultaneously in the cities of Sobral, Ceará, Brazil and in São Tomé and Príncipe on the west coast of Africa.[12] The result was considered spectacular news and made the front page of most major newspapers. It made Einstein and his theory of general relativity world famous. When asked by his assistant what his reaction would have been if general relativity had not been confirmed by Eddington and Dyson in 1919, Einstein famously made the quip: "Then I would feel sorry for the dear Lord. The theory is correct anyway." [13]
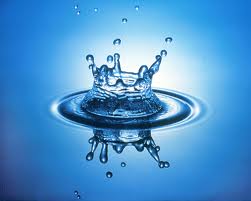
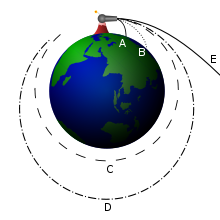
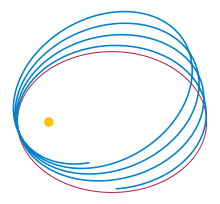
Newtonian (red) vs. Einsteinian orbit (blue) of a lone planet orbiting a star.
At its introduction in 1915, the general theory of relativity did not have a solid empirical foundation. It was known that it correctly accounted for the "anomalous" precession of the perihelion of Mercury and on philosophical grounds it was considered satisfying that it was able to unify Newton's law of universal gravitation with special relativity. That light appeared to bend in gravitational fields in line with the predictions of general relativity was found in 1919 but it was not until a program of precision tests was started in 1959 that the various predictions of general relativity were tested to any further degree of accuracy in the weak gravitational field limit, severely limiting possible deviations from the theory.
orrectly p
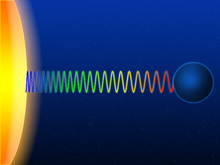
Schematic representation of the gravitational redshift of a light wave escaping from the surface of a massive body
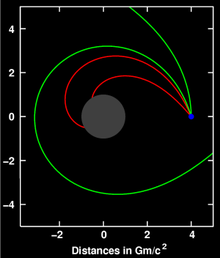
Deflection of light (sent out from the location shown in blue) near a compact body (shown in gray)
Einstein predicted the gravitational redshift of light from the equivalence principle in 1907, but it is very difficult to measure astrophysically (see the discussion under Equivalence Principle below). Although it was measured by Walter Sydney Adams in 1925, it was only conclusively tested when the Pound–Rebka experiment in 1959 measured the relative redshift of two sources situated at the top and bottom of Harvard University's Jefferson tower using an extremely sensitive phenomenon called the Mössbauer effect.[18][19] The result was in excellent agreement with general relativity. This was one of the first precision experiments testing general relativity.
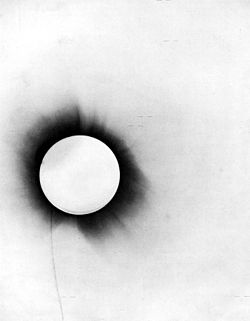
One of Eddington's photographs of the 1919 solar eclipse experiment, presented in his 1920 paper announcing its success
The early accuracy, however, was poor. The results were argued by some[14] to have been plagued by systematic error and possibly confirmation bias, although modern reanalysis of the dataset[15] suggests that Eddington's analysis was accurate.[16][17] The measurement was repeated by a team from the Lick Observatory in the 1922 eclipse, with results that agreed with the 1919 results[17] and has been repeated several times since, most notably in 1973 by a team from the University of Texas. Considerable uncertainty remained in these measurements for almost fifty years, until observations started being made at radio frequencies. It was not until the late 1960s that it was definitively shown that the amount of deflection was the full value predicted by general relativity, and not half that number. The Einstein ring is an example of the deflection of light from distant galaxies by more nearby objects.
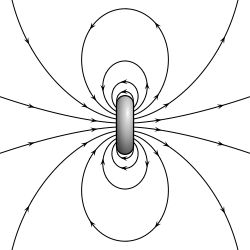
Magnetic Dipole
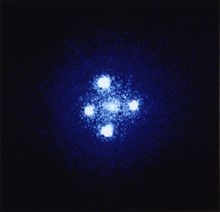
Einstein cross: four images of the same astronomical object, a quasar, produced by a gravitational lens
The Einstein Cross or Q2237+030 or QSO 2237+0305 is a gravitationally lensed quasar that sits directly behind ZW 2237+030, Huchra's Lens. Four images of the same distant quasar appear around a foreground galaxy due to strong gravitational lensing.
The quasar is located about 8 billion light years from Earth, while the lensing galaxy is located at a distance of 400 million light years
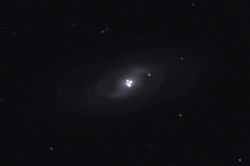
Huchra's Lens, a galaxy ZW 2237+030. It exhibits the phenomenon of gravitational lensing that was postulated by Albert Einstein when he realized that gravity would be able to bend light and thus could have lens-like effects.
Main article: Gravitational waves
One of several analogies between weak-field gravity and electromagnetism is that, analogous to electromagnetic waves, there are gravitational waves: ripples in the metric of spacetime that propagate at the speed of light.[66] The simplest type of such a wave can be visualized by its action on a ring of freely floating particles. A sine wave propagating through such a ring towards the reader distorts the ring in a characteristic, rhythmic fashion (animated image to the right).[67] Since Einstein's equations are non-linear, arbitrarily strong gravitational waves do not obey linear superposition, making their description difficult. However, for weak fields, a linear approximation can be made. Such linearized gravitational waves are sufficiently accurate to describe the exceedingly weak waves that are expected to arrive here on Earth from far-off cosmic events, which typically result in relative distances increasing and decreasing by 10 − 21 or less. Data-analysis methods routinely make use of the fact that these linearized waves can be Fourier decomposed.[68]
Some exact solutions describe gravitational waves without any approximation, e.g., a wave train traveling through empty space[69] or so-called Gowdy universes, varieties of an expanding cosmos filled with gravitational waves.[70] But for gravitational waves produced in astrophysically relevant situations, such as the merger of two black holes, numerical methods are presently the only way to construct appropriate models.