Gravity is the force which "pulls" an object back to earth. Understanding gravity is where my theory departs from modern gravitational theory.
Sir Isaac Newton came up with the first mathematical description of gravity, and it remained largely unchanged until Einstein came along and laid the framework for modern nuclear and atomic physics with the General Theory of Relativity. A lot has changed in the last 100 years since 1916.
Isaac Newton thought that (diag 1), if you fired a cannon from the top of a hill, parallel to the earth's surface, the object would fall back to earth (A). However, since it was falling at a constant speed (velocity), it would take a constant time to fall that distance (height). He deduced that if you could fire it with sufficient velocity it would travel further in that time before it touched the ground (B), and with sufficient velocity it would travel past the curvature of the earth, and continue falling (C) and remain in orbit. He thought more speed would achieve an elliptical orbit (D), and even more the projectile would escape gravity altogether (E). He was correct.
Speed of Gravity.
In the context of classical theories of gravitation, the speed of gravity is the speed at which changes in a gravitational field propagate. This is the speed at which a change in the distribution of energy and momentum of matter results in subsequent alteration, at a distance, of the gravitational field which it produces. In a more physically correct sense, the "speed of gravity" refers to the speed of a gravitational wave.
The speed of gravitational waves in the general theory of relativity is equal to the speed of light in vacuum, c.[1] Within the theory of special relativity, the constant c is not exclusively about light; instead it is the highest possible speed for any physical interaction in nature. Formally, c is a conversion factor for changing the unit of time to the unit of space.[2] This makes it the only speed which does not depend either on the motion of an observer or a source of light and/or gravity. Thus, the speed of "light" is also the speed of gravitational waves and any massless particle. Such particles include the gluon (carrier of the strong force), the photons that light waves consist of, and the theoretical gravitons which make up the associated field particles of gravity (a theory of the graviton requires a theory of quantum gravity, however).
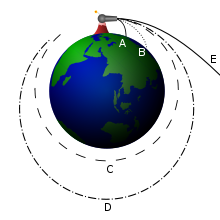
(Diag. 1) Isaac Newton's analysis of escape velocity. Projectiles A and B fall back to earth. Projectile C achieves a circular orbit, D an elliptical one. Projectile E escapes.
In physics, escape velocity is the speed at which the kinetic energy plus the gravitational potential energy of an object is zero [nb 1]. It is the speed needed to "break free" from a gravitational field without further propulsion.
For a spherically-symmetric body, escape velocity is calculated by the formula
where G is the universal gravitational constant (G=6.67×10-11 m3 kg-1 s-2), M the mass of the planet, star or other body, and r the distance from the centre of gravity.
In this equation atmospheric friction or air drag, is not taken into account. A rocket moving out of a gravity well does not actually need to attain escape velocity to do so, but could achieve the same result at walking speed with a suitable mode of propulsion and sufficient fuel. Escape velocity only applies to ballistic trajectories.
The term escape velocity is actually a misnomer, as the concept refers to a scalar speed which is independent of direction whereas velocity is the measurement of the rate and direction of change in position of an object.
To add more to this, we say an object has mass. What is the force which binds an object to the earth? It is gravity, bt we do not know how gravity does this.
If an object has mass, it has energy. The force, (momentum) of an object is equal to its mass multiplied by its velocity. For example, if we lift a mass of 1 kg (kilogram), 1 metre off the earth, it has potential energy. The energy was put there lifting away from the ground, wheter we did that slowly, or with an explosive.
In physics, a scalar is a simple physical quantity that is not changed by coordinate system rotations or translations (in Newtonian mechanics), or by Lorentz transformations or space-time translations (in relativity). This is in contrast to a vector. A related concept is a pseudoscalar, which is invariant under proper rotations but (like a pseudovector) flips sign under improper rotations.
Escape velocity is sometimes misunderstood to be the speed a powered vehicle, such as a rocket, must reach to leave orbit and travel through outer space. The quoted escape velocity is commonly the escape velocity at a planet's surface, but it actually decreases with altitude. It is the speed above which an object will depart on a ballistic trajectory, i.e. in free-fall, and never fall back to the surface nor assume a closed orbit. Such an object is said to "escape" the gravity of the planet.
A vehicle with a propulsion system can continue to gain energy and travel away from the planet, in any direction, at a speed lower than escape velocity so long as it is under power. If the vehicle's speed is below its current escape velocity and the propulsion is removed, the vehicle will assume a closed orbit or fall back to the surface. If its speed is at or above the escape velocity and the propulsion is removed, it has enough kinetic energy to "escape" and will neither orbit nor fall back to the surface.
Momentum
In classical mechanics, linear momentum or translational momentum (pl. momenta; SI unit kg•m/s, or, equivalently, N•s) is the product of the mass and velocity of an object:
Like velocity, linear momentum is a vector quantity, possessing a direction as well as a magnitude. Linear momentum is also a conserved quantity, meaning that if a closed system is not affected by external forces, its total linear momentum cannot change. Although originally expressed in Newton's second law, the conservation of linear momentum also holds in special relativity and, with appropriate definitions, a (generalized) linear momentum conservation law holds in electrodynamics, quantum mechanics, quantum field theory, and general relativity. In relativistic mechanics, non-relativistic linear momentum is further multiplied by the Lorentz factor.
Gravity will force it back to earth, and it will continue to accellerate (go faster) as it falls. A two kg object has twice the energy (momentum), that a 1 kg object does, falling the same distance. An object falling two metres has more than twice the the monentum that an object falling one metre does, because it is still accellerating.(more)